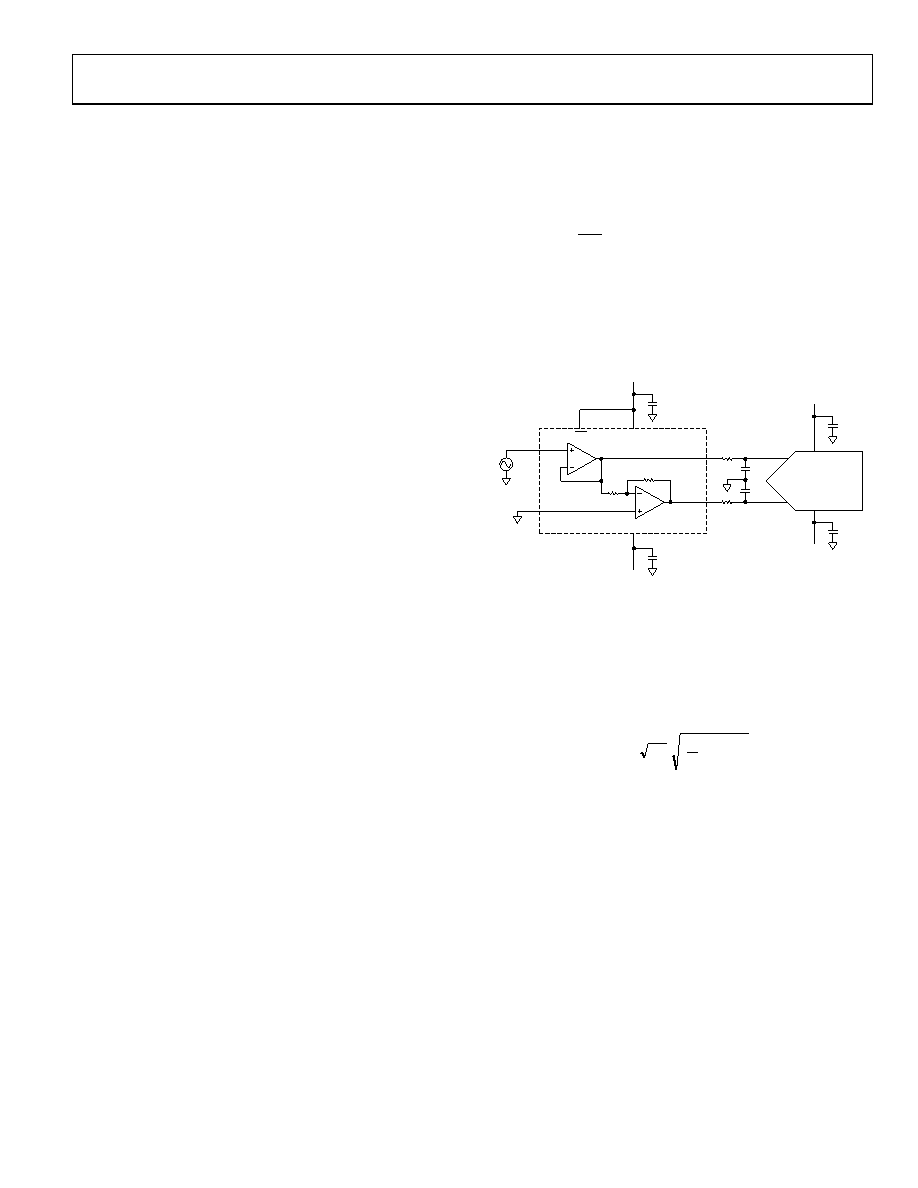
ADA4922-1
Rev. 0 | Page 17 of 20
Defining VIN as the voltage applied to the input pin, the
equations that govern the two signal paths are given in
Equation 12 and Equation 13.
VOUT+ = +VIN
(12)
VOUT = VIN + 2(REF)
(13)
When the REF voltage is set to the midswing level of the input
signal, the two output signals fall directly on top of each other
with minimal offset. Setting the REF voltage elsewhere results
in an offset between the two outputs. This effect is illustrated in
The best use of the REF pin can be further illustrated by
considering a single-supply example that uses a 10 V dc power
supply and has an input signal that varies between 2 V and 7 V.
This is a case where the midswing level of the input signal is not
at midsupply but is at 4.5 V. By setting the REF input to 4.5 V
and neglecting offsets, Equation 12 and Equation 13 are used to
calculate the results. When the input signal is at its midpoint of
4.5 V, VOUT+ is at 4.5 V, as is VOUT. This can be considered as a
type of baseline state where the differential output voltage is
zero. When the input increases to 7 V, VOUT+ tracks the input to
7 V and VOUT decreases to 2 V. This can be viewed as a positive
peak signal where the differential output voltage equals 5 V.
When the input signal decreases to 2 V, VOUT+ again tracks to
2 V, and VOUT increases to 7 V. This can be viewed as a negative
peak signal where the differential output voltage equals 5 V.
The resulting differential output voltage is 10 V p-p.
The previous discussion exposes how the single-ended-to-
differential gain of 2 is achieved.
INTERNAL FEEDBACK NETWORK POWER
DISSIPATION
While traditional op amps do not have on-chip feedback
elements, the ADA4922-1 contains two on-chip 600 Ω resistors
that comprise an internal feedback loop. The power dissipated
in these resistors must be included in the overall power dissipation
calculations for the device. Under certain circumstances, the
power dissipated in these resistors could be considerably more
than the device’s quiescent current. For example, on ±12 V
supplies with the REF pin tied to ground and OUT at 9 V dc,
each 600 Ω resistor carries 15 mA and dissipates 135 mW. This
is a significant amount of power and must therefore be included
in the overall device power dissipation calculations. For ac
signals, rms analysis is required.
DISABLE FEATURE
The ADA4922-1 includes a disable feature that can be asserted
to minimize power consumption in a device that is not needed
at a particular time. When asserted, the disable feature does not
place the device output in a high impedance or three-state
condition. The disable feature is asserted by applying a control
section for the high and low level voltage specifications.
DRIVING A DIFFERENTIAL INPUT ADC
The ADA4922-1 provides the single-ended-to-differential
conversion that is required to drive most high resolution ADCs.
Figure 50 shows how the ADA4922-1 simplifies ADC driving.
VIN
±10V
0.1
μF
–12V
HIGH VOLTAGE
HIGH RESOLUTION
ADC
VS–
VS+
DIS
056
81
-0
49
R
IN
8
OUT+
ADA4922-1
OUT–
4
5
6
73
REF
2
0.1
μF
–12V
0.1
μF
+12V
0.1
μF
+12V
R
C
Figure 50. Driving a Differential Input ADC
For example, consider the case where the input signal
bandwidth is 100 KHz and R = 41.2 Ω and C = 3.9 nF, as is
shown in
Figure 50, to form a single-pole filter with 3 dB
bandwidth of approximately 1 MHz. The ADA4922-1 output
noise (with zero source resistance) integrated over this
bandwidth appears at the ADC input and is calculated as
()
rms
μV
15
MHz
1
2
π
Hz
nV/
12
)
(
,
=
=
rms
V
dm
ADC
n,
(14)
The rms value of a 20 V p-p signal at the ADC input is 7 V rms,
yielding a SNR of 113 dB at the ADC input.