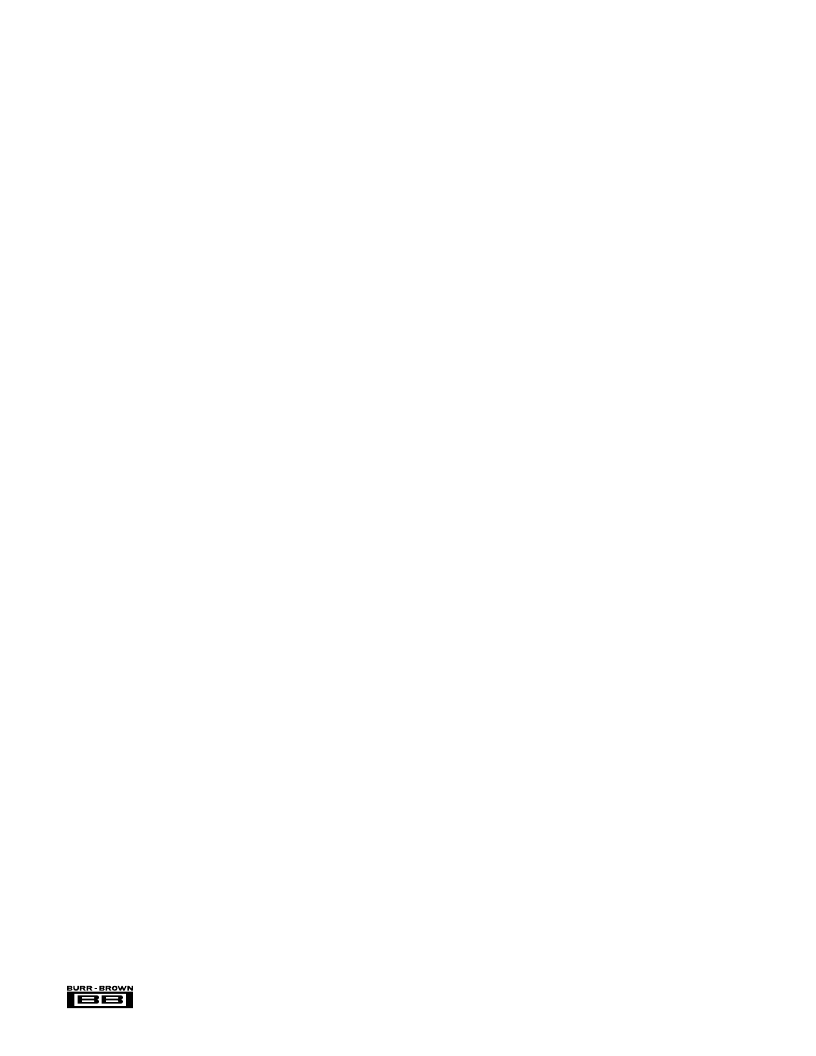
8
ISO254
SYNCHRONIZED OPERATION
ISO254 can be synchronized to an external signal source.
This capability is useful in eliminating troublesome beat
frequencies in multi-channel systems and in rejecting AC
signals and their harmonics. To use this feature, tie all sync
pins together or apply an external signal to the SYNC pin.
ISO254 can be synchronized to an external oscillator over
the range 1-1.4MHz (this corresponds to a modulation fre-
quency of 500kHz to 700kHz as SYNC is internally divided
by 2).
CARRIER FREQUENCY CONSIDERATIONS
ISO254 amplifiers transmit the signal across the ISO-barrier
by a duty-cycle modulation technique. This system works
like any linear amplifier for input signals having frequencies
below one half the carrier frequency, f
C
. For signal frequen-
cies above f
C
/2, the behavior becomes more complex. The
“Signal Response vs Carrier Frequency” performance curve
describes this behavior graphically. The upper curve illus-
trates the response for input signals varying from DC to f
C
/
2. At input frequencies at or above f
C
/2, the device generates
an output signal component that varies in both amplitude
and frequency, as shown by the lower curve. The lower
horizontal scale shows the periodic variation in the fre-
quency of the output component. Note that at the carrier
frequency and its harmonics, both the frequency and ampli-
tude of the response go to zero. These characteristics can be
exploited in certain applications.
It should be noted that for the ISO254, the carrier frequency
is nominally 400kHz and the –3dB point of the amplifier is
50kHz. Spurious signals at the output are not significant
under these circumstances unless the input signal contains
significant components above 200kHz.
When periodic noise from external sources such as system
clocks and DC/DC converters are a problem, ISO254 can be
used to reject this noise. The amplifier can be synchronized
to an external frequency source, f
EXT
, placing the amplifier
response curve at one of the frequency and amplitude nulls
indicated in the “Signal Response vs Carrier Frequency”
performance curve.
ISOLATION MODE VOLTAGE
Isolation Mode Voltage (IMV) is the voltage appearing
between isolated grounds GND1 and GND2. The IMV can
induce errors at the output as indicated by the plots of IMV
vs Frequency. It should be noted that if the IMV frequency
exceeds f
C
/2, the output will display spurious outputs in a
manner similar to that described above, and the amplifier
response will be identical to that shown in the “Signal
Response vs Carrier Frequency” performance curve. This
occurs because IMV-induced errors behave like input-
referred error signals. To predict the total IMR, divide the
isolation voltage by the IMR shown in “IMR vs Frequency”
performance curve and compute the amplifier response to
this input-referred error signal from the data given in the
“Signal Response vs Carrier Frequency” performance curve.
Due to effects of very high-frequency signals, typical IMV
performance can be achieved only when dV/dT of the
isolation mode voltage falls below 1000V/
μ
s. For conve-
nience, this is plotted in the typical performance curves
for the ISO254 as a function of voltage and frequency for
sinusoidal voltages. When dV/dT exceeds 1000V/
μ
s but
falls below 20kV/
μ
s, performance may be degraded. At rates
of change above 20kV/
μ
s, the amplifier may be damaged,
but the barrier retains its full integrity. Lowering the power
supply voltage below 15V may decrease the dV/dT to
500V/
μ
s for typical performance, but the maximum dV/dT
of 20kV/
μ
s remains unchanged.
Leakage current is determined solely by the impedance of
the barrier and transformer capacitance and is plotted in the
“Isolation Leakage Current vs Frequency” curve.
ISOLATION VOLTAGE RATINGS
Because a long-term test is impractical in a manufacturing
situation, the generally accepted practice is to perform a
production test at a higher voltage for some shorter time.
The relationship between actual test voltage and the continu-
ous derated maximum specification is an important one.
Historically, Burr-Brown has chosen a deliberately conser-
vative one: VTEST = (2
x
ACrms continuous rating) +
1000V for 10 seconds, followed by a test at rated ACrms
voltage for one minute. This choice was appropriate for
conditions where system transients are not well defined.
Recent improvements in high-voltage stress testing have
produced a more meaningful test for determining maximum
permissible voltage ratings, and Burr-Brown has chosen to
apply this new technology in the manufacture and testing of
the ISO254.
Partial Discharge
When an insulation defect such as a void occurs within an
insulation system, the defect will display localized corona or
ionization during exposure to high-voltage stress. This ion-
ization requires a higher applied voltage to start the
discharge and lower voltage to maintain it or extinguish it
once started. The higher start voltage is known as the
inception voltage, while the extinction voltage is that level
of voltage stress at which the discharge ceases. Just as the
total insulation system has an inception voltage, so do the
individual voids. A voltage will build up across a void until
its inception voltage is reached, at which point the void will
ionize, effectively shorting itself out. This action redistrib-
utes electrical charge within the dielectric and is known as
partial discharge. If, as is the case with AC, the applied
voltage gradient across the device continues to rise, another
partial discharge cycle begins. The importance of this
phenomenon is that, if the discharge does not occur, the
insulation system retains its integrity. If the discharge be-
gins, and is allowed to continue, the action of the ions and
electrons within the defect will eventually degrade any
organic insulation system in which they occur. The measure-
ment of partial discharge is still useful in rating the devices