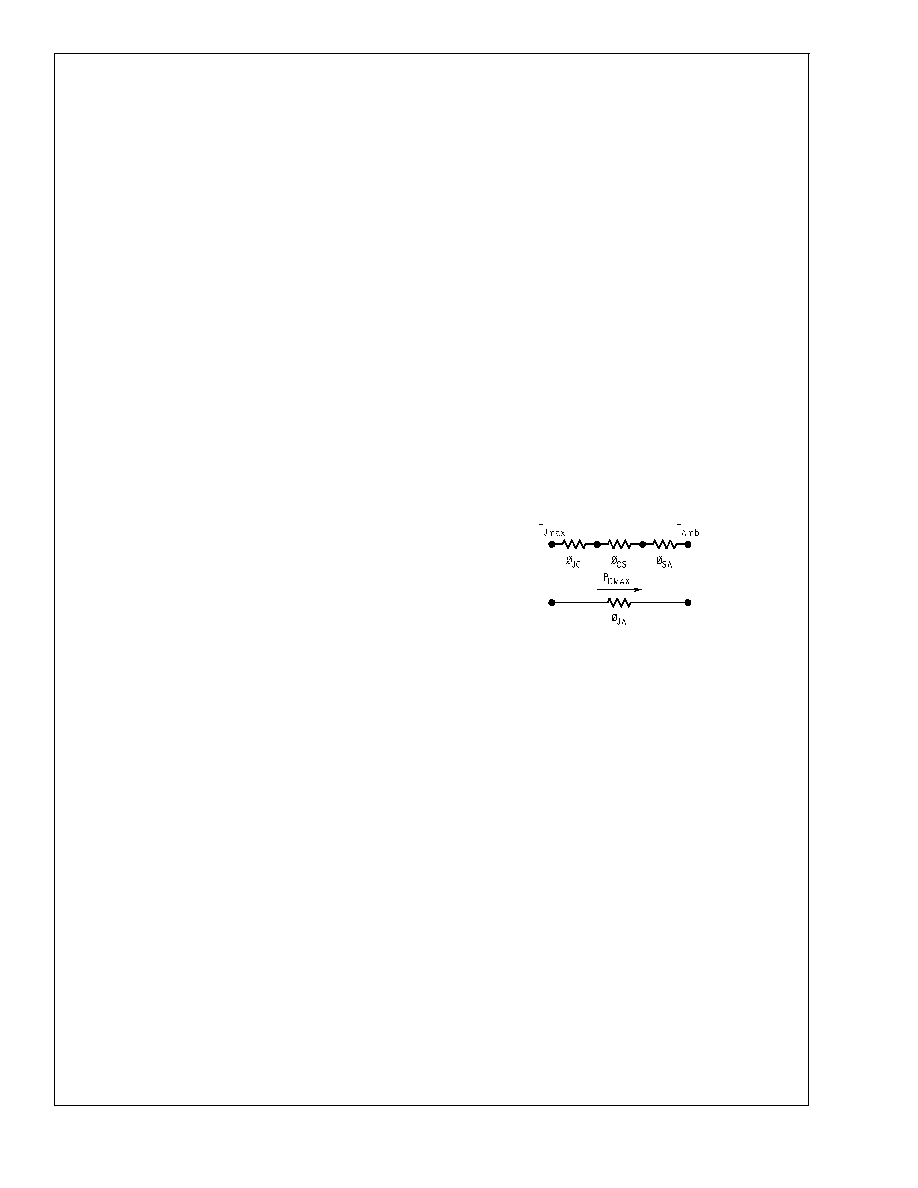
Application Information (Continued)
pulled out of mute mode. Taking into account supply line
fluctuations, it is a good idea to pull out 1mA per mute pin or
2 mA total if both pins are tied together.
UNDER-VOLTAGE PROTECTION
Upon system power-up, the under-voltage protection cir-
cuitry allows the power supplies and their corresponding
capacitors to come up close to their full values before turning
on the LM4766 such that no DC output spikes occur. Upon
turn-off, the output of the LM4766 is brought to ground
before the power supplies such that no transients occur at
power-down.
OVER-VOLTAGE PROTECTION
The LM4766 contains over-voltage protection circuitry that
limits the output current to approximately 4.0A
PK while also
providing voltage clamping, though not through internal
clamping diodes. The clamping effect is quite the same,
however, the output transistors are designed to work alter-
nately by sinking large current spikes.
SPiKe PROTECTION
The
LM4766
is
protected
from
instantaneous
peak-
temperature stressing of the power transistor array. The Safe
Operating graph in the Typical Performance Characteris-
tics section shows the area of device operation where
SPiKe Protection Circuitry is not enabled. The waveform to
the right of the SOA graph exemplifies how the dynamic
protection will cause waveform distortion when enabled.
Please refer to AN-898 for more detailed information.
THERMAL PROTECTION
The LM4766 has a sophisticated thermal protection scheme
to prevent long-term thermal stress of the device. When the
temperature on the die reaches 165C, the LM4766 shuts
down. It starts operating again when the die temperature
drops to about 155C, but if the temperature again begins to
rise, shutdown will occur again at 165C. Therefore, the
device is allowed to heat up to a relatively high temperature
if the fault condition is temporary, but a sustained fault will
cause the device to cycle in a Schmitt Trigger fashion be-
tween the thermal shutdown temperature limits of 165C and
155C. This greatly reduces the stress imposed on the IC by
thermal cycling, which in turn improves its reliability under
sustained fault conditions.
Since the die temperature is directly dependent upon the
heat sink used, the heat sink should be chosen such that
thermal shutdown will not be reached during normal opera-
tion. Using the best heat sink possible within the cost and
space constraints of the system will improve the long-term
reliability of any power semiconductor device, as discussed
in the Determining the Correct Heat Sink Section.
DETERMlNlNG MAXIMUM POWER DISSIPATION
Power dissipation within the integrated circuit package is a
very important parameter requiring a thorough understand-
ing if optimum power output is to be obtained. An incorrect
maximum power dissipation calculation may result in inad-
equate heat sinking causing thermal shutdown and thus
limiting the output power.
Equation (1) exemplifies the theoretical maximum power
dissipation point of each amplifier where V
CC is the total
supply voltage.
P
DMAX =VCC
2/2
π2R
L
(1)
Thus by knowing the total supply voltage and rated output
load, the maximum power dissipation point can be calcu-
lated. The package dissipation is twice the number which
results from
Equation (1) since there are two amplifiers in
each LM4766. Refer to the graphs of Power Dissipation
versus Output Power in the Typical Performance Charac-
teristics section which show the actual full range of power
dissipation not just the maximum theoretical point that re-
sults from
Equation (1).
DETERMINING THE CORRECT HEAT SINK
The choice of a heat sink for a high-power audio amplifier is
made entirely to keep the die temperature at a level such
that the thermal protection circuitry does not operate under
normal circumstances.
The thermal resistance from the die (junction) to the outside
air (ambient) is a combination of three thermal resistances,
θ
JC, θCS, and θSA. In addition, the thermal resistance, θJC
(junction to case), of the LM4766T is 1C/W. Using Thermal-
loy Thermacote thermal compound, the thermal resistance,
θ
CS (case to sink), is about 0.2C/W. Since convection heat
flow (power dissipation) is analogous to current flow, thermal
resistance is analogous to electrical resistance, and tem-
perature drops are analogous to voltage drops, the power
dissipation out of the LM4766 is equal to the following:
P
DMAX =(TJMAXTAMB)/θJA
(2)
where T
JMAX = 150C, TAMB is the system ambient tempera-
ture and
θ
JA = θJC + θCS + θSA.
Once the maximum package power dissipation has been
calculated using
Equation (1), the maximum thermal resis-
tance,
θ
SA, (heat sink to ambient) in C/W for a heat sink can
be calculated. This calculation is made using
Equation (3)
which is derived by solving for
θ
SA in Equation (2).
θ
SA = [(TJMAXTAMB)PDMAX(θJC +θCS)]/PDMAX
(3)
Again it must be noted that the value of
θ
SA is dependent
upon the system designer’s amplifier requirements. If the
ambient temperature that the audio amplifier is to be working
under is higher than 25C, then the thermal resistance for the
heat sink, given all other things are equal, will need to be
smaller.
SUPPLY BYPASSING
The LM4766 has excellent power supply rejection and does
not require a regulated supply. However, to improve system
performance as well as eliminate possible oscillations, the
LM4766 should have its supply leads bypassed with
low-inductance capacitors having short leads that are lo-
cated close to the package terminals. Inadequate power
supply bypassing will manifest itself by a low frequency
oscillation known as “motorboating” or by high frequency
instabilities. These instabilities can be eliminated through
multiple bypassing utilizing a large tantalum or electrolytic
capacitor (10F or larger) which is used to absorb low fre-
quency variations and a small ceramic capacitor (0.1F) to
prevent any high frequency feedback through the power
supply lines.
DS100928-52
LM4766
www.national.com
11