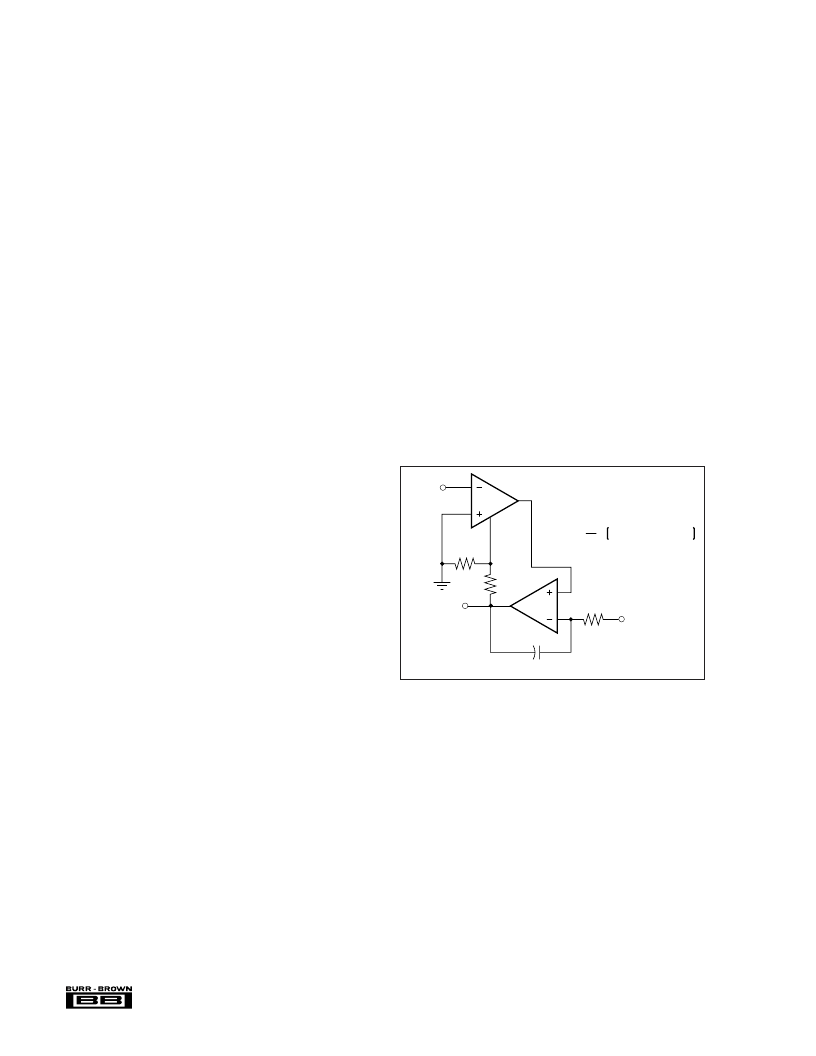
VCA610
10
STABILIZED WEIN-BRIDGE OSCILLATOR
Adding Wein-bridge feedback to the above AGC amplifier
produces an amplitude-stabilized oscillator. Shown in
Figure 8, this alternative requires the addition of just two
resistors (R
W1
, R
W2
) and two capacitors (C
W1
, C
W2
).
Connecting the feedback network to the amplifier’s
noninverting input introduces positive feedback to induce
oscillation. The feedback factor displays a frequency depen-
dence due to the changing impedances of the C
W
capacitors.
As frequency increases, the decreasing impedance of the
C
W2
increases the feedback factor. Simultaneously, the de-
creasing impedance of the C
W1
decreases this factor.
Analysis shows that the maximum factor occurs at f =
1/2
π
R
W
C
W
, making this the frequency most conducive to
oscillation. At this frequency the impedance magnitude of
C
W
equals R
W
and inspection of the circuit shows that this
condition produces a feedback factor of 1/3. Thus, self-
sustaining oscillation requires a gain of three through the
amplifier. The AGC circuitry establishes this gain level.
Following initial circuit turn on, R
1
begins charging C
H
negative, increasing the amplifier gain from its minimum.
When this gain reaches three, oscillation begins at f =
1/2
π
R
W
C
W
and R
1
’s continued charging effect makes the
oscillation amplitude grow. This growth continues until that
amplitude reaches a peak value equal to V
R
. Then, the AGC
circuit counteracts the R
1
effect, controlling the peak ampli-
tude at V
R
by holding the amplifier gain at a level of three.
Making V
R
an AC signal, rather than a DC reference,
produces amplitude modulation of the oscillator output.
LOW-DRIFT WIDEBAND LOG AMP
The VCA610 can be used to provide a 250kHz (–3dB) log
amp with low offset voltage and low gain drift.
The exponential gain control characteristic of the VCA610
permits simple generation of a temperature-compensated
logarithmic response. Enclosing the exponential function in
an op amp feedback path inverts this function, producing the
log response. Figure 9 shows the practical implementation
of this technique. A DC reference voltage, V
R
, sets the
VCA610 inverting input voltage. This makes the amplifier’s
output voltage V
OA
= – GV
R
where G = 10
-2 (Vc + 1)
.
A second input voltage also influences V
OA
through control
of gain G. The feedback op amp forces V
OA
to equal the
input voltage V
IN
connected at the op amp inverting input.
Any difference between these two signals drops across R
3
,
producing a feedback current that charges C
C
. The resulting
change in V
OL
adjusts the gain of the VCA610 to change
V
OA
. At equilibrium, V
OA
= V
IN
= –V
R
10
-2 (Vc +1)
. The op
amp forces this equality by supplying the gain control
voltage V
C
= R
1
V
OL
/(R
1
+ R
2
). Combining the last two
expressions and solving for V
OL
yields the circuit’s logarith-
mic response.
V
OL
= – (1 + R
2
/R
1
) [1 + 0.5LOG (–V
IN
/V
R
)]
Examination of this result illustrates several circuit charac-
teristics. First, the argument of the Log term, –V
IN
/V
R
,
reveals an option and a constraint. In Figure 9, V
R
represents
a DC reference voltage. Optionally, making this voltage a
second signal produces log-ratio operation. Either way, the
Log term’s argument constrains the polarities of V
R
and V
IN
.
These two voltages must be of opposite polarities to ensure
a positive argument. This polarity combination results when
V
R
connects to the inverting input of the VCA610. Alter-
nately, switching V
R
to this amplifier’s noninverting input
removes the minus sign of the log term’s argument. Then,
both voltages must be of the same polarity to produce a
positive argument. In either case, the positive polarity re-
quirement of the argument restricts V
IN
to a unipolar range.
The above V
OL
expression reflects a circuit gain introduced
by the presence of R
1
and R
2
. This feature adds a convenient
scaling control to the circuit. However, a practical matter
sets a minimum level for this gain. The voltage divider
formed by R
1
and R
2
attenuates the voltage supplied to the
V
C
terminal by the op amp. This attenuation must be great
enough to prevent any possibility of an overload voltage at
the V
C
terminal. Such an overload saturates the VCA610’s
gain control circuitry, reducing the amplifier’s gain. For the
feedback connection of Figure 9, this overload condition
permits a circuit latch. To prevent this, choose R
1
and R
2
to
ensure that the op amp can not possibly deliver more than
2.5V to the V
C
terminal.
FIGURE 9. Driving the Gain Control Pin of the VCA610 with
a Feedback Amplifier Produces a Temperature-
Compensated Log Response.
R
1
470
VCA610
R
2
330
V
OL
V
R
–10mV
OPA620
V
IN
V
OA
= –G V
R
C
C
50pF
R
3
100
V
OL
= – 1 + R
1 + 0.5 Log (–V
IN
/V
R
)
2
R
1
V
C
LOW-DRIFT WIDEBAND EXPONENTIAL AMP
A common use of the Log amp above involves signal
companding. The inverse function, signal expanding, re-
quires an exponential transfer function. The VCA610 pro-
duces this latter response directly as shown in Figure 10. DC
reference V
R
again sets the amplifier’s input voltage and the
input signal V
IN
now drives the gain control point. Resistors
R
1
and R
2
attenuate this drive to prevent overloading the
gain control input. Setting these resistors at the same values
as in the preceding Log amp produces an exponential ampli-
fier with the inverse function of the Log amp.