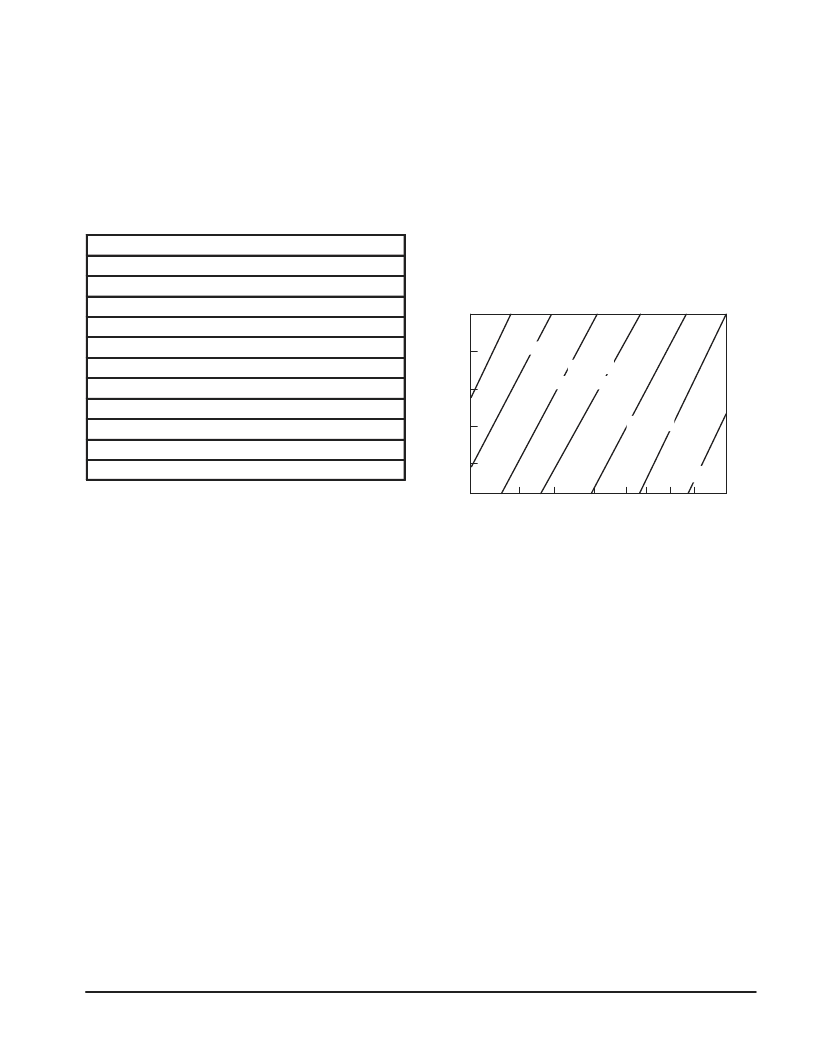
1–19
Motorola Sensor Device Data
For More Information On This Product,
Go to: www.freescale.com
The failure mechanisms that may affect a sensor or actuator
will be discussed along with the contributors and acceleration
means. Failure mechanisms of interest during media testing
of semiconductor MEMS devices are shown in Table 1. MEMS
applications may involve disposable applications such as a
blood pressure monitor whose lifetime is several days.
General attributes to consider during testing include: lifetime
expectations, cost target, quality level, size, form, and
functionality.
Table 1. Typical Failure Mechanisms for
Sensors and Actuators [6–10]
Failure Mechanism
Uniform Corrosion
Localized Corrosion
Galvanic Corrosion
Silicon Etching
Polymer Swelling or Dissolution
Interfacial Permeability
Adhesive Strength
Fatigue Crack Initiation
Fatigue Crack Propagation
Environment Assisted Cracking
Creep
Methods for performing media compatibility testing to
determine the potential for the various failure mechanisms will
be presented. Attributes of the testing need to be well
understood so that proper assessment of failure and lifetime
approximation can be made. The lifetime modeling is key for
determination of the ability of a sensor device to perform its
intended function. Reliability modeling and determination of
activation energies for the models will provide the customer
with an understanding of the device performance. The
definition of an electrical failure can range from catastrophic,
to exceeding a predetermined limit, to just a small shift. The
traditional pre to post electrical characterization (before and
after the test interval) can be enhanced by in situmonitoring.
In situmonitoring may expose a problem with a MEMS device
during testing that might have gone undetected once the
media or another environmental factor is removed. This is a
common occurrence for a failure mechanism, such as
swelling, that may result in a shift in the output voltage of the
sensor. Response variables during environmental testing can
include: electrical, visual, analytical, or physical characteristics
such as swelling or weight change.
DEFINITIONS & UNDERLYING CAUSES
The definition of a media compatible pressure sensor is as
follows:
The ability of a pressure sensor to perform its specified
electromechanical function over an intended lifetime in the
chemical, electrical, mechanical, and thermal environments
encountered in a customer’s application.
The key elements of the definition are perform, function,
lifetime, environment, and application. All of these elements
are critical to meet the media compatibility needs. The
underlying causes of poor media compatibility is the hostile
environment and permeability of the environment. The
environment may consist of media or moisture with ionics,
organics, and/or aqueous solutions, extreme temperatures,
voltage, and stress.
Permeability is the product of diffusivity and solubility.
Contributors to permeability include materials (e.g. polymeric
structures), geometry, processing, and whether or not the
penetration is in the bulk or at an interface. The environment
can also accelerate permeation if a concentration gradient,
elevated temperature and/or pressure exist. An example of
material dependence of permeation is shown in Figure 2.
Organic materials such as silicone can permeate 50% of the
relative moisture from the exterior within minutes where
inorganic materials such as glass takes years for the same
process to occur.
Figure 2. Permeation relationship for various materials.
MIN
HR
DAY
MO YR
10
YR
100
YR
–5
–4
–3
–2
–1
–6
SILICONES
FLUORO–CARBONS
10–6
10–8
10–10
10–12
10–14
10–16
L
PERMEABILITY (g/cm–s–torr)
TIME FOR PACKAGE INTERIOR TO REACH
50% OF EXTERIOR HUMIDITY *
METALS
EPOXIES
GLASSES
* Richard K. Traeger, “Nonhermiticity of Polymeric Lid Sealants,
IEEE Transactions on Parts, Hybrids, and Packaging, Vol. PHP–13,
No. 2, June 1977.
Gasoline and aqueous alkaline solutions represent two
relatively diverse applications that are intended for use with a
micromachined pressure sensor. The typical automotive
temperature range is from –40
°
to 150
°
C. This not only makes
material selection more difficult but also complicates the
associated hardware to perform the media related testing [11].
A typical aqueous alkaline solution application would be found
in the appliance industry. This industry typically has a
narrower temperature extreme then the automotive market,
but the solutions and the level of ions provide a particular
challenge to MEMS device reliability.
Gasoline contains additives such as: antiknock,
anti–preignition agents, dyes, antioxidants, metal
deactivators, corrosion inhibitors, anti–icers, injector or
carburetor detergents, and intake valve deposit control
additives [12]
.
To develop a common test scheme for the
liquid, a mixture table was developed for material testing in
gasoline/methanol mixtures. The gasoline/methanol mixtures
developed were intended for accelerated material testing with
a gasoline surrogate of ASTM Fuel Reference “C” (50%
toluene and 50% iso–octane) [13]. Material testing is
performed with samples either immersed in the liquid or
exposed to the vapor over the liquid. The highly aromatic Fuel
F
Freescale Semiconductor, Inc.
n
.